The latest turning technology for titanium parts
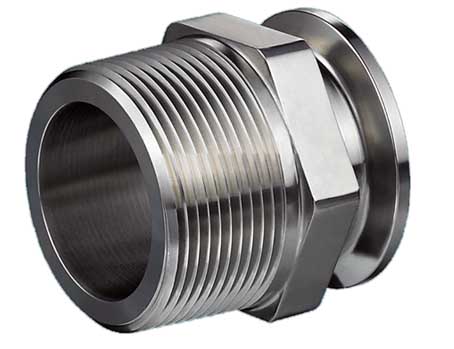
However, the turning of titanium alloy parts is very difficult, which makes the machining technicians daunting. They believe that the super-performance of titanium alloys greatly reduces the "ability" of its machinability, making its cutting process extremely challenging.
Although this view makes sense, it is not comprehensive. This article explores the latest turning strategy for titanium alloys. It is helpful for machining technicians to process difficult-to-machine materials for a wide range of applications (such as racing parts, underwater breathing apparatus, etc.) and to apply new cutting techniques.
BillHeadland, a senior project specialist at RTI International Metals, a titanium alloy manufacturer, pointed out. Although many processing plants regard titanium processing as a fearful path, in fact titanium alloys cover a wide range of products, you must know which one you are processing. Titanium alloys have many grades, some of which are difficult to machine, while others are not. Commercially pure titanium (CP grade) is a non-alloy material commonly used in the manufacture of medical parts, heat exchangers and eyeglass frames. CP grades have excellent corrosion resistance and are relatively easy to process. However, compared with other titanium alloys, its strength is very low, and it is sticky and soft.
After the addition of the alloy to pure titanium, the crystal phase (crystal structure) is changed, and the properties and machinability of the material are also changed. The α-titanium alloy and the quasi-α titanium alloy contain additives such as nickel, aluminum, and vanadium. The machinability of these intermediate grades of titanium alloys is quite good. Alpha-beta titanium grades may contain more aluminum and vanadium. The industrial mainstream titanium alloy Ti6Al4V is an alpha-beta titanium alloy grade containing about 6% aluminum and 4% vanadium. Ti6Al4V and its variants account for about 50%-70% of the titanium alloys currently used.
A beta grade titanium alloy with iron, chromium and other ingredients added is one of the most difficult grades to process. Due to its high fracture toughness and excellent resistance to high cycle fatigue, the mechanical properties of the beta grades are similar to Hastelloy's nickel-based alloys and similar materials. A typical application example is the manufacture of a lightweight spring that is used to trigger a collapsible tail of an underwater launch tactical missile.
Various titanium alloys exhibit different cutting properties. Some people think that the time required to machine a Ti6Al4V workpiece is usually three times that of a steel piece; Others say that the time required to machine a Ti5553 beta workpiece is twice the time required to machine a Ti6Al4V workpiece.
In turning, the most important characteristic of titanium alloys is poor thermal conductivity. The high temperature generated during cutting is difficult to be absorbed by the workpiece and collects on the cutting edge of the tool. Excessive heat causes a chemical reaction between the cutting edge and the chip and produces crater wear.
Titanium alloys also have a tendency to work harden, so it is important to remove the metal by shear rather than extrusion. In addition, although titanium alloys have high strength, they also have a low modulus of elasticity. This means that titanium alloys are relatively more elastic and easier to leave the cutting edge than other materials (especially during light load cutting). Taking into account these characteristics of titanium alloys, in order to successfully achieve the turning of titanium alloys, the key is to achieve a balance of cutting speed, feed rate and depth of cut.
Cutting speed is the primary factor affecting the generation of cutting heat. Sandvik Coromant's turning expert Stefan Gyllengahm spent three and a half years developing tool grades for tool manufacturers. During this time, he performed a Ti6Al4V cutting test in the laboratory, and the results showed that the cutting speed must be carefully selected: In some cases, when the cutting speed is increased by 10%-15%, the tool life will be reduced from 40 to 6 pieces, which indicates that the cutting speed is adjusted too much. He also found that when turning at a cutting speed that does not shorten the tool life, if the feed rate is increased, a critical temperature that is detrimental to the tool life is reached because there is a limit range of excessive heat.
Tool geometry plays a key role in controlling chip form for heat dissipation. The wider and thinner chips enlarge the contact area between the chip being formed and the cutting edge, thereby reducing the accumulation of heat at the cutting edge. If the cutting chips are thinner and generate less heat, the cutting speed can be faster.
For example, when roughing is performed using a C-type (80°) diamond blade having a standard lead angle of -5°. The chip thickness is approximately equal to the feed rate; The use of a square blade with a lead angle of 45° allows the cut metal (and cutting heat) to spread along the longer cutting edge. The circular blade theoretically maximizes this concept (but only when the depth of cut is small). According to Gyllengahm, very thin chips are usually obtained with a round insert when the depth of cut is small. However, considering the round blade having a circular cutting 12.7mm effective lead angle decreases when the depth of cut is greater than 2mm, it is preferable to use square inserts. Because at the same depth of cut, the square blade still has a 45° lead angle.
Bill Skoretz is the manager of the processing division of Patriot Forge. The company offers a wide range of raw materials, from low-alloy steels and stainless steels to special grades of aluminum alloys and titanium alloys, and sometimes to titanium alloy parts for the company's customers. When discussing the challenges of turning titanium alloys, he focused on the experience of coolant jets for turning mills. This corrosion-resistant titanium alloy grade is abrasive. Due to the resilience of titanium alloys, a positive tool geometry must be used and the shape of the tool head must be emphasized. If the bottom or front corner of the tool is too small, the tool will start to generate tension, which causes a lot of problems. Therefore, an optimum balance must be found between the positive geometrical angle of the tool head and the support of the cutting edge.
Skoretz describes the development of cutting tools from a historical perspective. Before the development of cemented carbide tools, high-speed steel tools were mainly used to cut steel. The advent of carbide tools makes it possible to use a positive geometric angle, but the machine must have sufficient power. Negative rake angle inserts only allow the titanium alloy material to fold or squash, making it difficult to remove it. But he also warned that if the positive rake angle of the blade is too large, it will also form a tensile force on the titanium alloy material. Therefore, a balance must be found between compressive and tensile stresses. He mentioned that in the past a blade having a T-shaped land with a 0.1 mm or 0.13 mm surface was used on the cutting edge. "Mainly for the safety of the blade, you can't use a sharp cutting edge because it won't last long." He also uses oil-based cutting fluids in his machining, but mainly uses its lubricating properties rather than cooling capacity. Other processing plants also have different methods of turning titanium because there are different solutions for the removal of any material.
Other processing plants also have different titanium turning methods, as there are different solutions for the removal of any titanium material.
Rayco Machine has 30% of its business dealing with racing parts, many of which are expensive titanium parts. Because the top racing team is willing to pay extra for lightweight, high-strength parts. In order to keep their cars at the minimum weight allowed by the rules, while still maintaining control over the distribution of their overall body weight. For example, reducing the mass of the rotating and non-suspended parts (such as the wheel and brake components) can effectively improve acceleration and handling performance. Rayco's turning titanium parts include parts that run between the brake caliper and the rotor.
Rayco Machine has 30% of its business dealing with racing parts, many of which are expensive titanium parts. Because the top racing team is willing to pay extra for lightweight, high-strength parts. In order to keep their cars at the minimum weight allowed by the rules, while still maintaining control over the distribution of their overall body weight. For example, reducing the mass of the rotating and non-suspended parts (such as the wheel and brake components) can effectively improve acceleration and handling performance. Rayco's turning titanium parts include parts that run between the brake caliper and the rotor.
Like Skoretz, Rayco's president, Greg Cox, said that in order to successfully turn titanium alloys, a balanced approach is needed. He believes that when selecting cutting parameters, it is very important that the workpiece material cannot be pushed during processing, otherwise work hardening is likely to occur, which causes great trouble for processing. The processing parameters commonly used by Rayco are: Cutting speed 120sfm, feed rate 0.13-0.20mm.
The depth of cut is also important. Again, moderate depth of cut is the best choice. Rayco's maximum depth of cut is 0.8-1mm. Rayco's titanium parts can be processed in batches of up to 200 pieces, but most are between 5-20 pieces. Cox expressed, Rayco company also continued to improve the process, but care must be taken when it comes to cutting parameters, because titanium is quite expensive and can not act recklessly so as not to lead to parts obsolescence. The price of titanium alloys rose very quickly, rising from $47/lb to $68/lb last year. The high price of titanium alloys also tightens the inventory of workpiece materials.
Scott Holland, general manager of the R&D and manufacturing division of diving equipment manufacturer Atomic Aquatics, said. When machining underwater respirators with titanium, “we always try to continuously improve machining efficiency to reduce machining time, machine more parts and extend tool life.” But when they tried to machine more parts, it was the tool that suddenly broke. Therefore, Holland hopes to achieve an optimal balance point, but this balance is not just numbers and procedures. Holland has nearly a decade of experience in titanium machining, and he relies on observing the shape of the workpiece and the tool and listening to cutting noise to master this balance. Holland said that processing titanium alloys can also be relatively simple. “If you use a sharp tool and change the tool at your own estimated time, you can only machine titanium in a limited range. You can try it your way, but it doesn't necessarily work. There are certain rules in the processing of titanium alloys. If you master these rules, you can be handy. ”
The increasing use of titanium alloys has driven the development of cutting technology with a focus on efficient turning of titanium alloys. Chris Mills, Senior Manager, Process Management and Technical Support, Sandvik Coromant, emphasizes. Chemical wear is the primary tool failure mechanism when turning titanium alloys, while cutting high temperatures accelerate chemical wear.
When the hot swarf is drawn across the rake face of the tool, the cobalt is actually "pushed out" from the blade. Mills describes how to reduce the cutting temperature in two steps: The first step is to use a tool geometry design (such as a square or round blade with a 45° lead angle) to reduce the chip thickness to reduce the cutting temperature. Reducing the resulting crater wear, ultimately enabling higher feed rates and longer tool life; The second step is to use a high pressure coolant with a special morphology. This coolant not only has high pressure, but also exhibits a very precise laminar spray pattern. A "water wedge" can be formed between the chip and the rake face of the blade to hold the chip so that contact with the rake face is minimized to avoid crater wear.
Sandvik's Jetbreak cooling system is available at 1000-3000 psi with a 1.27 mm diameter nozzle and standard emulsified coolant. It not only has a cooling effect, but also generates lift for lifting the chips, which reduces the friction and temperature between the chips and the rake face. Use this system to increase cutting speed by 50%.
Mills describes the effect of combining the two cooling strategies described above: When machining titanium alloy with optimized cutting parameters (cutting speed: 40m/min. feed rate: 0.3mm/rev) with a -5° lead angle CNMG insert, the tool life is about 20 minutes;
When machining with a round blade or a square blade with a 45° lead angle. The cutting speed can be increased to 50-60m/min, the feed rate is increased to 0.4mm/rev, and similar tool life can be maintained. Since more workpiece material can be removed at the same time, the productivity can be doubled with only a square blade. If you use a high-pressure cooling system, you can increase the cutting speed by another 50%.
The high pressure coolant must be transported through the passage inside the machine shaft (instead of the external pipe). The coolant flows through a dedicated connector on the Sandvik Capto quick change tool chuck, which is controlled by Capto. This high-pressure cooling system can be easily installed when the machine is installed.
For regular machining titanium parts (particularly large and expensive aircraft parts) workshop, productivity increased by 50%, they are worth the investment to buy a dedicated machine tool chuck and a high-pressure cooling system installed.
This high-pressure cooling system has a unique advantage in turning titanium alloys because it does not produce crater wear as much as other workpiece materials.